Section 2 –
Canadian Subatomic Physics Research Plan
With an established track record; ongoing and newly developing international partnerships; valuable local research facilities such as TRIUMF, SNOLAB and the Perimeter Institute; and significant recent support for the development of experimental infrastructure, the Canadian community is poised to capitalize on a number of science opportunities over the coming five years, and the decade beyond. This section outlines the emerging science opportunities and the enabling technologies that will support progress. The research program is then presented in the form of a multi-dimensional portfolio of projects that will help the Canadian community maximize its scientific impact, training opportunities, and benefits to society. The existing portfolio, and emerging opportunities lead to a series of Science Recommendations.
Science Opportunities for Canada
Unique features of the Canadian subatomic physics research ecosystem position the community well to seize emerging scientific opportunities, with the goal of maximizing scientific impact, training opportunities and benefits to society. In particular, Canada has significant research infrastructure in TRIUMF, SNOLAB, and the Perimeter Institute. In addition, there are active community organizations and flexible funding structures to support new scientific initiatives. Canada also has an excellent standing as a trusted international partner, and the community has the capacity to train additional highly qualified personnel (HQP).
Several scientific opportunities exist in the coming years, and these are highlighted for each of the science drivers in the following sections.
Science Driver —
Higgs, physics at the electroweak scale, and beyond
In the coming decade, there will be unique opportunities to thoroughly explore the Higgs sector, study the physics of electroweak symmetry breaking, and search for new physics at the energy frontier, with significant prospects for a wide range of exciting new results. Dedicated searches for unconventional physics signatures will also offer significant discovery potential. There will be several opportunities to indirectly explore new regions of multi-TeV physics through measurements of known and rare physics processes at an unprecedented level of precision, providing complementary possibilities for observing hints of new physics. It is also likely that by the end of the decade, the situation with respect to persisting anomalies in the B-physics sector pointing to possible lepton flavour violation will be clarified.
Building on its expertise and past investments, Canada is well-positioned to pursue further scientific opportunities through its participation and leadership, for example, in the broad physics programs of ATLAS and Belle II, dedicated searches for new physics at MATHUSLA and MoEDAL, and precision programs such as those of MOLLER and NA62. There is also an active Canadian theory community engaged in interpreting new data and pointing to potential signatures of new physics. Finally, there is the opportunity to advance detector and accelerator R&D synergistically with contributions to the HL-LHC, the development of Chiral Belle, the International Linear Collider and the Future Circular Collider projects.
Science Driver —
Fundamental Symmetries and Observed Asymmetries
Exploration of the fundamental symmetries in subatomic physics and their violation will continue to be at the forefront of searches for new physics phenomena and principles, providing powerful and complementary sensitivities. Opportunities exist for probing symmetries to a new level of precision by exploiting a variety of different techniques.
Within the landscape of possibilities, the combined Canadian expertise in atomic, nuclear, particle physics and accelerator research offers unique opportunities for Canada to play a scientifically leading role world-wide. TRIUMF can become a global centre for tests of CP and T violation via EDM searches, with the start of operations for the Canadian-led TUCAN project, the FrEDM experiment, and the development of the RAMS facility using radium monofluoride and francium silver molecules. New tests of parity violation can be achieved with FrPNC, studies of beta decays with ISAC and TRINAT at TRIUMF, and the launch of Nab operations at ORNL. Canadians are also well-positioned to play a significant role in future tests of the electroweak symmetry structure and the running of the Weinberg angle with the development of MOLLER, along with Belle II and the possible Chiral Belle upgrade. Spectroscopic tests of anti-hydrogen with significantly improved precision will be feasible with ALPHA-3 and ALPHA-g, and the deployment of Canadian-led HAICU. Tests of lepton flavour universality will continue with NA62 at CERN, and the potential development of PIONEER. Tests of neutrino properties and CP violation will continue with long-baseline neutrino experiments such as T2K and will move to the next generation of precision with Hyper-K and DUNE operations. Tests of lepton number violation via neutrinoless double beta decay can be further explored with next generation experiments such as nEXO, LEGEND or other detectors complementing the existing SNO+ program at SNOLAB.
Science Driver —
Neutrino Properties
The coming decade should be another exciting one for neutrino physics. At its conclusion, it is likely that the mass hierarchy will be determined, and the search for CP violation will be well underway, potentially yielding conclusive results. At the same time, searches for 0vββ will have continued to push forward, and will likely have achieved sensitivity to span the inverted hierarchy parameter space. Measurements of astrophysical neutrinos will have continued to inform us about the highest energy processes in the cosmos, and neutrinos will have further illuminated our understanding of the workings of the Sun and the interior of the Earth. Research in neutrino physics continues to move forward with vigour, and new breakthrough discoveries are a distinct possibility.
Canada is well-positioned to play a leading role in all of these scientific achievements, with the development of Hyper-K and DUNE, the evolution of IceCube and potential development of POne, and the primacy of SNOLAB as the preferred location for tonne-scale experiments such as nEXO and LEGEND.
Science Driver —
Dark Matter and Potential Dark Sectors
Significant breakthroughs in our understanding of the nature of dark matter are possibly within reach in the next decade. Experiments designed to directly detect the presence of dark matter in our galactic HALO are likely to achieve significant increases in sensitivity through the continuing development of experimental techniques. As experimental sensitivity approaches the important background from solar and atmospheric neutrinos (the so-called “neutrino floor”), possible new directions include the development of experiments capable of exploring diverse mass scales, dark matter electron scattering, and directional capability in the reconstruction of dark matter interactions. In the coming decade, several accelerator-based projects aiming to produce dark matter and particles related to a possible dark sector should also have acquired significant data allowing further direct tests of this paradigm. The search for dark matter through observation of its annihilation signatures will be pursued using a variety of observatories of increasing sensitivity. The Canadian astroparticle theory community is also well-positioned to play a synergistic role in this effort.
Canada has a bright future in the search for dark matter and is in an excellent position to seize these scientific opportunities. The Cryogenic Underground Test Experiment (CUTE) infrastructure at SNOLAB will enable early exploitation of the SuperCDMS crystals for physics results. By the end of the decade, the SuperCDMS experiment at SNOLAB, will have explored a large swath of parameter space for low mass WIMPs and approach sensitivity to the solar and atmospheric neutrino background. The DEAP collaboration has joined the Global Argon Dark Matter Collaboration with the goal of running DarkSide-20k at the Gran Sasso Underground Laboratory (LNGS) in Italy and then a multi-hundred tonne detector ARGO with SNOLAB being the preferred location. The PICO experiment is expected to continue to improve its leading sensitivity in the spin-dependent WIMP sector, while the new SBC experiment will adapt this exciting new scintillating bubble-chamber technology to the search for low mass WIMPs. The NEWS-G experiment is also poised to make interesting contributions to the low mass regime and will explore directional sensitivity using a novel composite central anode in its detector. High-precision accelerator-based dark sector searches will be carried out at ATLAS, Belle II, NA62, MoEDAL and MOLLER. The DarkLight experiment is also preparing a technical design report for use of the intense ARIEL electron beam on a thin target at TRIUMF to explore dark sectors. Canadians will also take part in the commissioning and operation of a demonstrator for the future MATHUSLA project. Indirect searches for dark matter annihilation will continue at IceCube.
Science Driver —
New Physical Principles and Structures
Canadian theorists pursuing a deeper understanding of the foundations of subatomic physics have established global stature across a range of research sub-fields. This presents opportunities for progress in several topical areas. Canadian theorists are pursuing basic questions about the underlying structure of quantum field theory, including improved methods for calculating scattering amplitudes, the geometric structures that underpin them, and the consistency constraints on allowed quantum field theories in the strong coupling regime. The role of quantum information theory in quantum field theories is another growing area of theoretical activity across Canada; through the AdS/CFT correspondence, this work is driving an understanding of the black hole information paradox, Hawking radiation, and quantum gravity more generally. Holography (the AdS/CFT correspondence and its extensions) also continues to present opportunities to advance our understanding of strongly coupled gauge theories, with ongoing progress modeling nuclear physics such as the quark-gluon plasma, and hydrodynamics. Further research opportunities within string theory target an explanation for various features of the Standard Model, as well as properties of the vacuum, such as a cosmological constant or dark energy. This also connects to further avenues for progress, as theoretical developments in quantum field theory may provide new ideas about the very early history of the universe and its initial conditions. Cosmological observations promise in turn to provide more information about the high-energy nature of subatomic physics, including the properties of dark matter. The universality of formal theoretical tools used in subatomic physics also presents opportunities for connections to other areas, including astrophysics, condensed matter physics, and quantum computing.
Formal theoretical efforts are primarily motivated by the goal of identifying underlying structures that can systematize and extend our theoretical understanding of fundamental physics. However, over the long term, formal research will continue to feed back to more phenomenological areas of subatomic physics to inform our understanding of many of the other science drivers, as it has in the past. Canadian theorists are strongly positioned to advance formal theory on a number of fronts, providing new directions for phenomenological and experimental subatomic research over the long term.
Science Driver —
Hadron Properties and Phases
In the coming decade, new experimental capabilities and advances on a range of theoretical fronts will help to shed light on nucleon structure and hadron properties.
Upgraded detectors for GlueX at JLab and at MAMI will extend the reach in precision and available nuclear targets. The proposed JLab Eta Factory (JEF) involves a significant upgrade to the GlueX base instrumentation, enhancing the energy and position resolution, and allowing for unprecedented precision in exotic hybrid meson searches. The Solenoidal Large Intensity Detector (SoLID) at JLab will study Generalized Parton Distributions, which can provide a tomographic 3D picture of the nucleon. Neutron spin polarizability measurements will be possible with a combination of measurements on He and He at MAMI, with the development of an active, high-pressure helium target.
Looking ahead, the future Electron-Ion Collider (EIC) is the only North American collider to be constructed for the foreseeable future and it is on Canada’s doorstep. The new opportunities at the EIC will make it possible to achieve a transformational understanding of the dynamical system of quarks and gluons. There is significant synergy between the EIC and 12 GeV JLab program, with a rich and diverse set of experiments capable of precisely studying QCD, from the nature of the finite temperature many-body problem, to mapping the transition from hadronic to partonic degrees of freedom. Canadian researchers are involved in all these projects, from forefront theoretical activities to the development of enabling technologies for the EIC like crab cavities, and are poised to make significant discoveries about hadron structure.
Science Driver —
Nuclear Structure
Developing a predictive understanding of nuclei and their interactions requires a wide variety of complementary experiments and theoretical tools. The coming decade will see the start of operations of new-generation infrastructure that will enable a systematic study of nuclear properties and patterns, potentially opening up a window to new and unexpected phenomena.
In Canada, the ARIEL facility and the CANREB project at TRIUMF will come online, promising a tripling of beam-time and extending the physics capability and reach for nuclear structure research. Canadian scientists will also continue to play a decisive role globally by contributing to the development of unique instruments and leading physics programs at RIBF (Japan), FAIR (Germany) and FRIB (USA).
The development of new ab initio theory, for both nuclear structure and interactions, is also Integral to this program. The synergy between experiment and theory, in terms of designing the most sensitive experiments and the feedback on the theoretical framework, will be crucial to shape a path towards the overarching goal of the field: a predictive standard model of nuclei.
Science Driver —
Cosmic Formation of Nuclei
The next decade will offer new scientific opportunities in studying heavy element synthesis due to the significant increase in the infrastructure for radioactive beams worldwide, combined with multi-messenger observations of neutron star mergers. For example, it will be possible to directly study the key reactions and short-lived nuclei required to understand the reaction pathways of explosive astrophysical events.
Canada is uniquely positioned to assume a leading role in these investigations with the start of operations for the ARIEL and CANREB facilities at TRIUMF. To fully exploit TRIUMF’s future beam capacity, various extensions to and upgrades of existing experimental capabilities are planned. For examples, a LaBr3 array is being planned that aims to achieve a ten fold increase in gamma tagging sensitivity of DRAGON. To enable new directions in reaction cross section measurements, especially with 3,4He, an active target time projection chamber (EXACT-TPC) is also being planned.
Offshore infrastructure will also offer new scientific opportunities. For example, higher intensities of rare nuclei will extend the reach in studying exotic decays with, for example, highly-charged ions in storage rings or beta-delayed multi-neutron emitters at GSI/FAIR. Measurement of the neutron skin thickness of neutron-rich nuclei, relevant for exploring the equation of state of asymmetric nuclear matter, is also planned at GSI/FAIR and at FRIB.
Future developments in ab initio theory promise to extend the reach to high-mass nuclei utilizing exascale computing power and the development of quantum computing and algorithmic capabilities. In the modeling of compact object mergers a variety of new developments are planned including molecular dynamics simulations.
In the longer term, the installation at TRIUMF of a low-energy storage ring with a neutron generator is being explored. This infrastructure project could provide a unique capacity for directly measuring the neutron capture cross sections of rare isotopes.
Opportunities Arising from Synergies with Other Fields
In the coming decade, knowledge acquired in other research fields may also help to advance our understanding of the subatomic physics science drivers. Examples include:
- Future developments in astronomy and astrophysics; e.g. potential gravitational wave signatures of early particle cosmology, potential signatures of dark matter in a number of future ground-based and satellite observatories, developments in the simulation of galaxy structure and formation, and multi-messenger observations of compact object mergers that could provide insight into the equation of state of high density matter.
- Next generation experiments measuring the cosmic microwave background will achieve a significant increase in precision in constraining the nature of neutrinos, dark matter, and dark sectors.
- Developments in the technology of quantum sensing and computing, and theoretical aspects of quantum condensed matter are occurring rapidly and are likely to open further opportunities in exploring the subatomic physics science drivers; some examples will be outlined below.
Likewise, there are opportunities for future subatomic physics research outcomes to have an impact on other related research fields:
- An experimental measurement of the absolute neutrino mass scale could have direct implications in cosmology.
- Precise measurements of new nuclear properties and rates will enable better understanding and modeling of processes in stellar astrophysics.
- Developments in accelerator and detector technology are likely to open further opportunities for research in other fields; examples include the development of
- new medical physics diagnostics and treatment.
- applications supporting green technologies.
- space systems designed for deep space exploration.
- imaging and tomography instruments for material science.
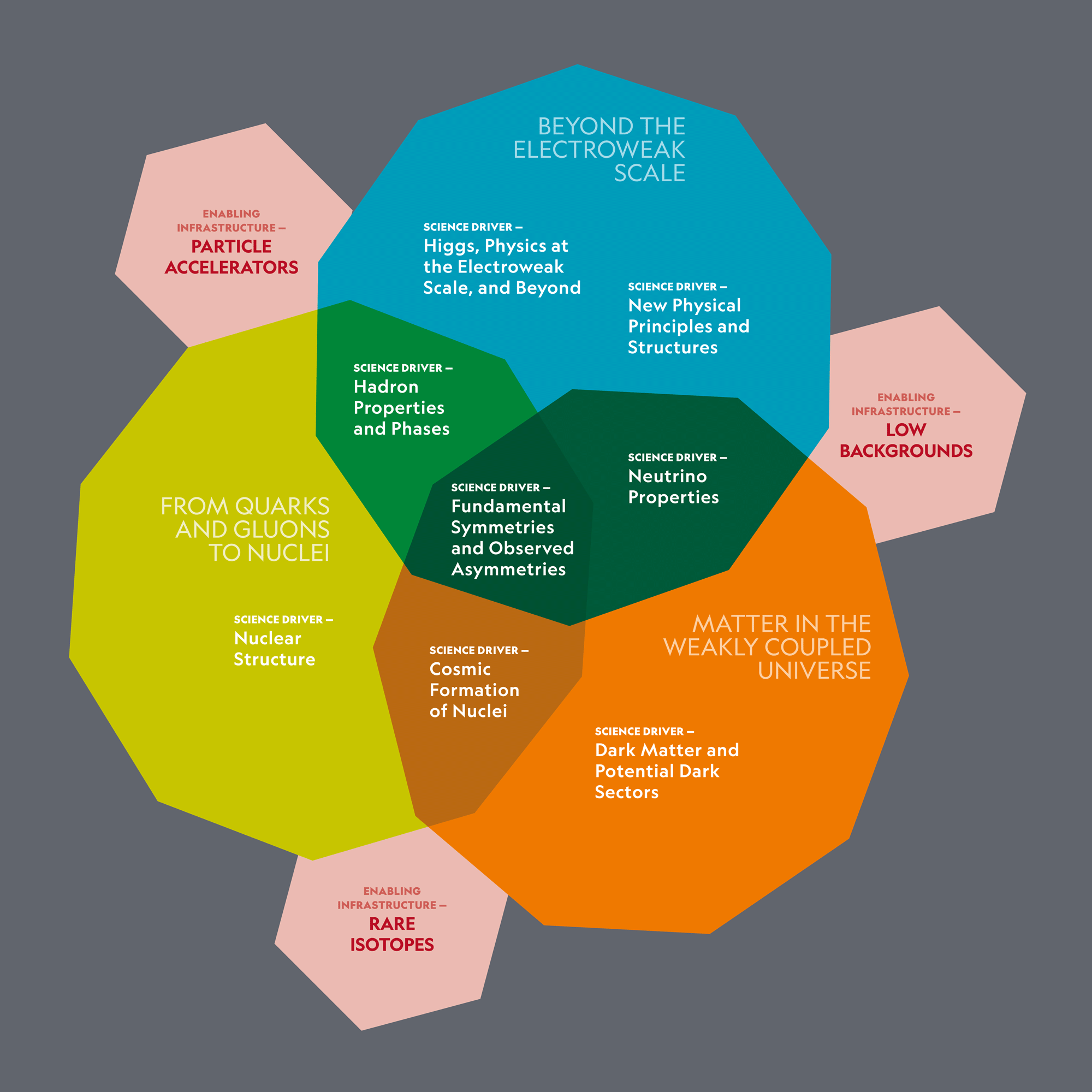
Infrastructure and Enabling Technologies
Progress for each of the science drivers depends on many factors, but a variety of infrastructure and enabling technologies are critical for both experimental and theoretical developments. These are described below along with their application to each of the science drivers.
Specialized Infrastructure
Subatomic physics research is enabled by and critically relies on the development and availability of unique infrastructure, as shown in Figure 3.
Particle Accelerators
Particle accelerator infrastructure continues to be a driving force in the development of experimental particle and nuclear physics, and is an enabling infrastructure for almost all the science drivers. The field of accelerator physics is in turn synergistic with subatomic physics itself, driven by many of the science goals, but with many broader applications. Accelerator infrastructure with significant Canadian access and involvement includes: TRIUMF in Canada, LHC at CERN, JPARC and KEK in Japan, Fermilab, JLab, RHIC and the future EIC in the US, and MAMI in Germany. Necessary developments for this type of infrastructure in support for subatomic physics research include:
- Accelerator R&D, focused on the Superconducting Radio-frequency (SRF) technology.
- Development of high-luminosity beams (neutrinos, electrons, neutrons, kaons, pions, ions, or antimatter) for precision measurements.
- Development of polarized beams of different species.
- Progress in understanding beam physics allowing precision control for operation at higher intensity or efficiency.
Further development of accelerator technology also involves the study of novel acceleration techniques, such as beam acceleration in plasmas.
Rare Isotopes
Studies of nuclear structure, including those relevant for astrophysical processes that formed the elements, are enabled by rare isotope beams. TRIUMF is a world-class facility that provides unique isotope beams at low energy and near Coulomb barrier energies, with a comprehensive set of detectors for analysis. The completion of ARIEL will significantly grow TRIUMF’s capacity to deliver isotope beams.
Complementary capabilities exist at the following offshore rare isotopes facilities with relativistic beams: Facility for Rare Isotope Beams (FRIB) in the USA, Facility for Antiproton and Ion Research (FAIR) in Germany, and the RI Beam Factory (RIBF) at RIKEN in Japan.
Some of the important areas in which development of this infrastructure is required include beam targetry, beam transport and detector capabilities.
Low Backgrounds
Measurements of rare processes and those requiring high precision depend critically on low background facilities. This enabling infrastructure is critical for experimental progress in searches for dark matter and neutrino properties, and for high-precision tests of fundamental symmetries. Facilities with significant Canadian access and involvement include: SNOLAB in Canada, Gran Sasso in Italy, and WIPP in the US. Specific developments in this type of infrastructure required to support subatomic physics include:
- Development and production of ultra-clean materials.
- Development of improved material assay techniques.
- Precisely controlled and monitored electromagnetic fields, beam properties and beam dynamics, as well as high and precisely measured beam polarization.
- Specialized high-precision detectors.
Enabling Tools and Emerging Technologies
Developments in the area of instrumentation, data analysis, theory, and computing enable tool-driven revolutions that can open the door to future discoveries. It is therefore important to maintain and further strengthen a research and development environment that stimulates and supports innovation in these areas.
Detector R&D
Detector R&D is key to enabling future discoveries. As such, it is important that the community sustains and promotes a diversified portfolio of both generic R&D and R&D activities with a focus on solving the known technological challenges of the next generation of experiments. Instrumentation development for subatomic physics is both a driver for, and a beneficiary of, progress made in other areas of subatomic physics and other fields of science and industry, and technology innovation often emerges from these synergies. Examples of detector developments include:
- radiation-hard semi-conductor devices for tracking detectors in future collider experiments.
- high-performance and novel photodetectors.
- low threshold nuclear and electron recoil detection technologies, including quantum sensors.
- capability for measuring combined features such as those associated with the development of 4D tracking and 5D imaging.
Digital Research Infrastructure
Subatomic physics research requires access to state-of-the-art digital research infrastructure with high computing capacity, petabyte-scale storage, and high-speed network connectivity between different computing sites and research centres world-wide, in order to manage large shared datasets. In the coming years, such digital research infrastructure will be provided and managed in Canada by CANARIE and the New Digital Research Infrastructure Organization (NDRIO) currently being established. As highlighted in the Tri-Agency Research Data Management Policy, “research data management (RDM) is a necessary part of research excellence”, and in this context, it is important to note that the needs of subatomic physics research include not just general storage of data from experiments but also long-term archival (data preservation) storage.
Analysis, Theory, and Computing
Tools for the analysis of ever-more complex data sets, and the development of the theoretical framework to understand the basic laws of subatomic physics need to evolve in concert with experimental techniques. Subatomic physics has driven the development of many analysis technologies, including Monte Carlo simulation for modelling signal and backgrounds, and the use of machine learning algorithms for data analysis. Meanwhile, developments in phenomenological modelling, computational technologies and in our theoretical understanding, point to new connections and synergies. Specific developments include:
- Machine Learning methodologies in triggering, simulation, and data analysis.
- Quantum Computing and quantum algorithms for reconstructing, simulating, and analysing data from large particle physics experiments.
- New amplitude calculation techniques, that have allowed higher-order analysis of Standard Model backgrounds at colliders.
- New theoretical connections and synergies with other fields, e.g. the use of quantum information tools in analyzing the physics of black holes.
Research Portfolio
In order to maximize the impact of Canada’s subatomic physics program, accounting for all the opportunities outlined above, the following overarching objectives for the subatomic physics research plan have been identified:
- Focus effort on the most relevant research problems.
- Fully exploit Canada’s unique facilities, competitive advantages, and past investments.
- Partner in leading international research projects and deliver on commitments.
- Maintain capacity and flexibility to explore and develop new scientific opportunities.
- Fully engage HQP in all aspects of scientific research to maximize training outcomes.
The LRP Committee sees value for the community in formulating the research plan as a portfolio of research projects, where an optimum balance among various dimensions would maximize the likelihood of scientific impact according to the objectives above while minimizing risk. The axes (or dimensions) of the portfolio include the following:
- Canadian scientific specialization vs breadth.
- Experimental project lifecycle (R&D and construction vs operation and science output).
- Guaranteed scientific output vs high-risk/high-reward.
- Project timeline.
- Theory vs experiment.
With an optimal balance across these dimensions, the portfolio provides a community-led vision of the future subatomic physics science priorities and the most efficient means to tackle them. It also conveys both new science opportunities, inter-connections between research sub-fields, and the need for resources on the longer timelines that are now common for large-scale subatomic physics projects.
A schematic representation of the current subatomic physics research portfolio, in relation to the science drivers, is shown in Figure 4.
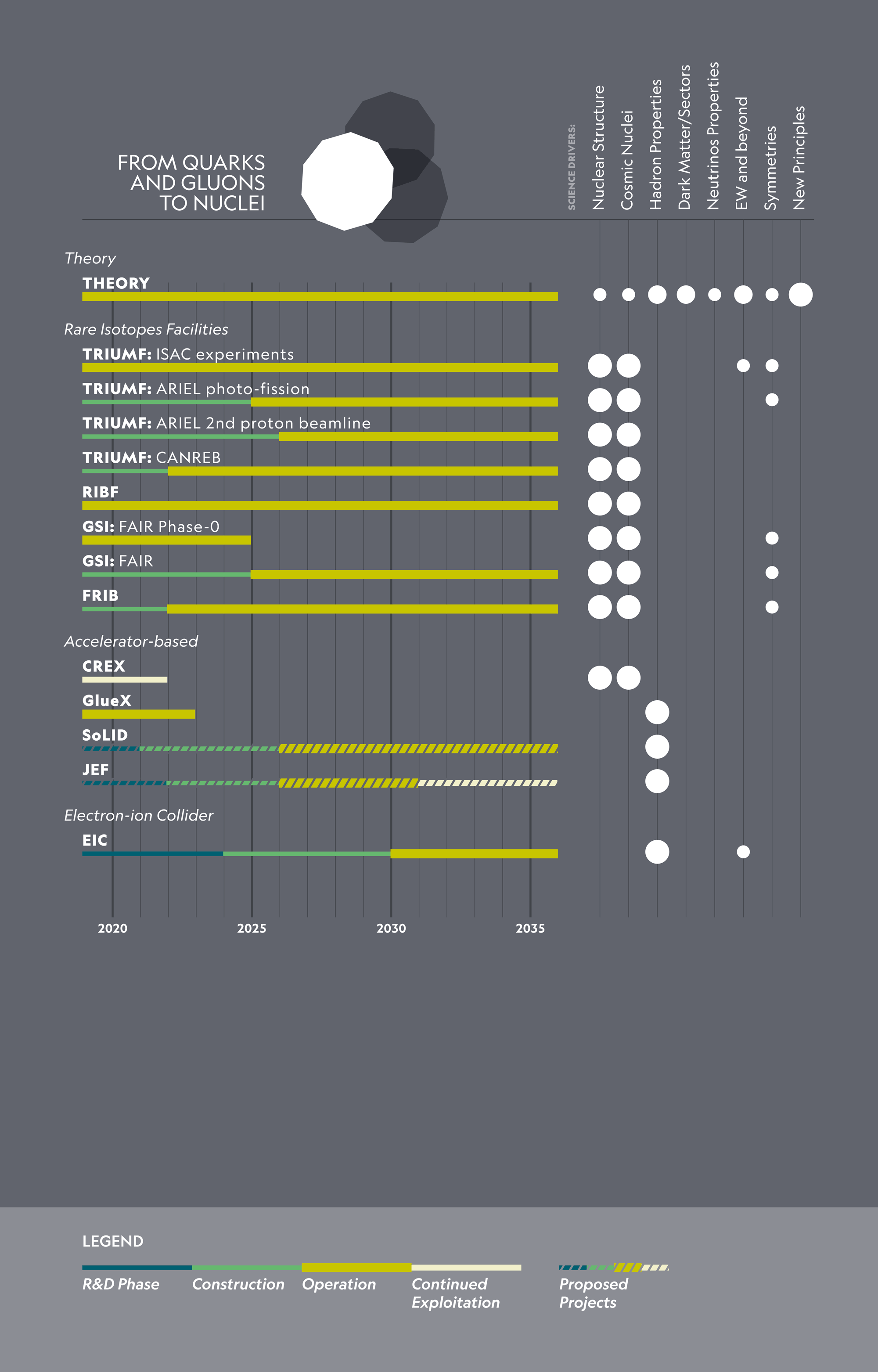
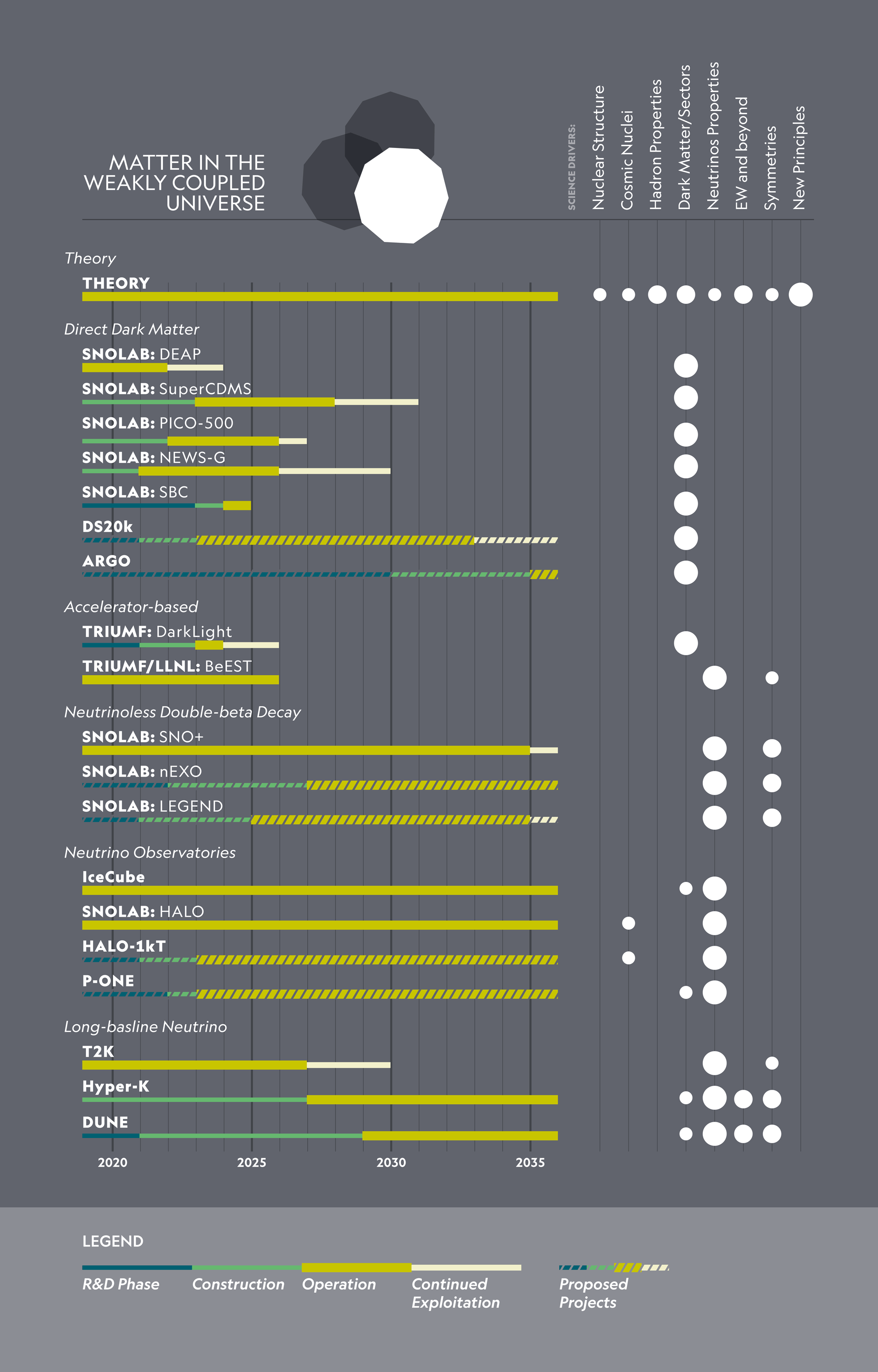
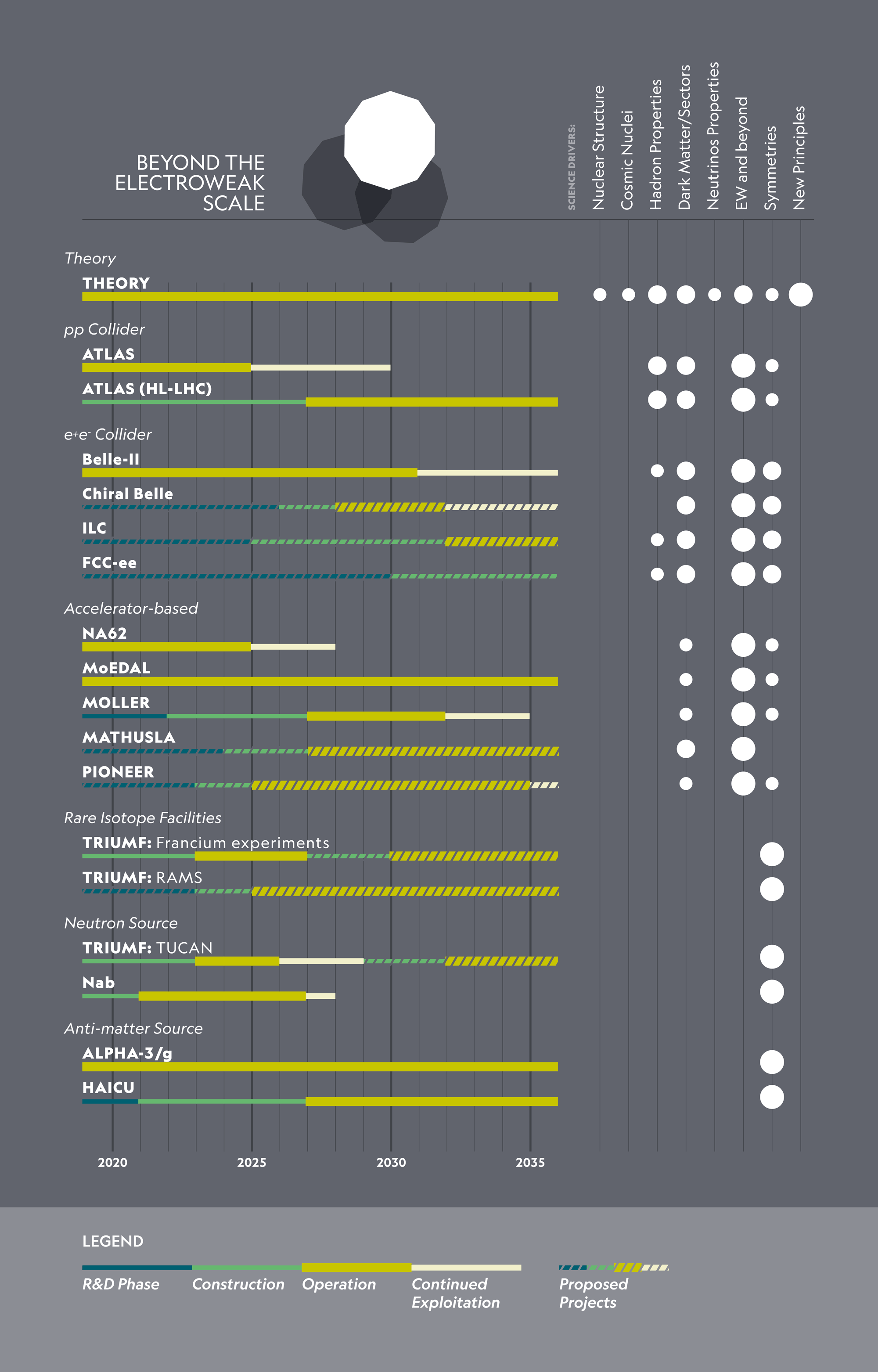
Science Recommendations
The LRP Committee has developed a number of Science recommendations that build upon the ongoing research activities, as exhibited in the portfolio, and the emerging research opportunities outlined above. These recommendations are described below.
Canada is fortunate to have several world-class subatomic physics research labs and facilities. These include the experimental infrastructure for nuclear physics at TRIUMF, and one of the deepest underground low-background facilities for neutrino and dark matter physics at SNOLAB. In addition, the Perimeter Institute is one of the premier centres in the world for theoretical physics. These centres conduct research, and also act as focal points to stimulate collaboration with the global community. Significant investments have been made over the past decade at TRIUMF, including the development of ARIEL, and at SNOLAB in bringing new experimental space online. It is a high priority that these investments be fully capitalized upon to pursue the new science opportunities that they enable.
Science Recommendation
1 —
Canadian Infrastructure
We recommend fully capitalizing upon the unique science opportunities provided by the SNOLAB and TRIUMF infrastructure, and by the Perimeter Institute, in pursuit of the science drivers.

Subatomic physics relies critically on both theoretical and experimental developments, as it seeks to understand the basic physical laws. It is vital for the health of the community that theoretical work is pursued along two paths, one that is fully collaborative with nuclear and particle experiment to interpret and understand data and point to new opportunities, and the other to explore new theoretical structures and seek to understand aspects of nature that fall outside the Standard Model, such as the quantum nature of gravity, black holes, and early cosmology. The Canadian theory community has traditionally had significant success in both these directions, and requires support to enable continuing global impact.
Science Recommendation
2 —
Theory Programs
Critical mass and research breadth are vital for the theory community in Canada, to maximize the future impact of subatomic physics research. We recommend strong support for theoretical subatomic physics research over the next decade, both to explore new purely theoretical directions and to support the synergistic interaction between subatomic theory and experiment.

The Canadian subatomic physics community has achieved considerable global impact by carefully identifying experimental projects which address the leading scientific questions. The opportunities for the ongoing and future program were outlined above, and the current suite of projects fills out the portfolio represented schematically in Figure 4. These considerations lead to the following recommendations for the experimental program.
Science Recommendation
3 —
Experimental Programs
A broad experimental program is required to address the scientific drivers of subatomic physics research. We recommend pursuit of the following high-priority scientific directions.
- From quarks and gluons to nuclei — The future program should explore the structure of hadrons and nuclei using rare isotope and accelerator-based facilities. It should include the full exploitation of TRIUMF, offshore electron beam and rare isotope beam (RIB) facilities, and a future electron-ion collider.
- Matter in the weakly coupled universe — The future program should incorporate the search for dark matter using complementary direct and indirect techniques, including via multi-ton scale direct detection. The future program should include the further exploration of neutrino properties via neutrinoless double-beta decay experiments, long baseline experiments and neutrino observatories.
- Beyond the electroweak energy scale — The future program should study matter and its interactions at increasingly higher energy scales, including the exploitation of a future Higgs factory and energy frontier collider, as well as high-precision indirect techniques.
This scientific program is currently implemented through Canadian leadership in a set of flagship projects identified based on their potential scientific payoff, Canadian core expertise, the level of community engagement, opportunities for the scientific and technological training of the next generation, and Canadian investments to date:
Flagship projects with broad physics outcomes | Flagship projects with strategic physics outcomes | |
---|---|---|
From quarks and gluons to nuclei | TRIUMF ARIEL-ISAC experiments, EIC | JLab 12 GeV program, Offshore RIB experiments |
Matter in the weakly coupled universe | T2K/HK, IceCube, SNO+ | DEAP, PICO-500, SuperCDMS |
Beyond the electroweak energy scale | ATLAS(LHC/HL-LHC), Belle II | ALPHA/HAICU, MOLLER, TUCAN |
We recommend the support of these projects and also those initiatives within the scientific program, with the potential for high impact, that are under development or may be developed in the coming years. Potential future projects with ongoing development activities and their timelines are listed in the research portfolio presented in Figure 4.

With the longer term outlook through to 2036 in mind, it is important to emphasize that the development of future projects depends critically on the ability of the community to explore, develop and assess the utility of new technologies in a manner that is not tied too closely to their final project application. Canada’s involvement in new domestic and international projects requires the capacity to develop and utilize new technologies, and early engagement maximizes opportunities for leadership, intellectual property development and subsequent training. However, such generic R&D is not fully supported within the current project-based funding ecosystem, and we highlight the need for additional support for this critical aspect that supports the long-term development and progress of subatomic physics.
Science Recommendation
4 —
R&D Activities
We recommend the support of R&D activities for the future development of particle accelerators and detector technology, and the development and use of emerging technologies including novel computational and analysis tools.
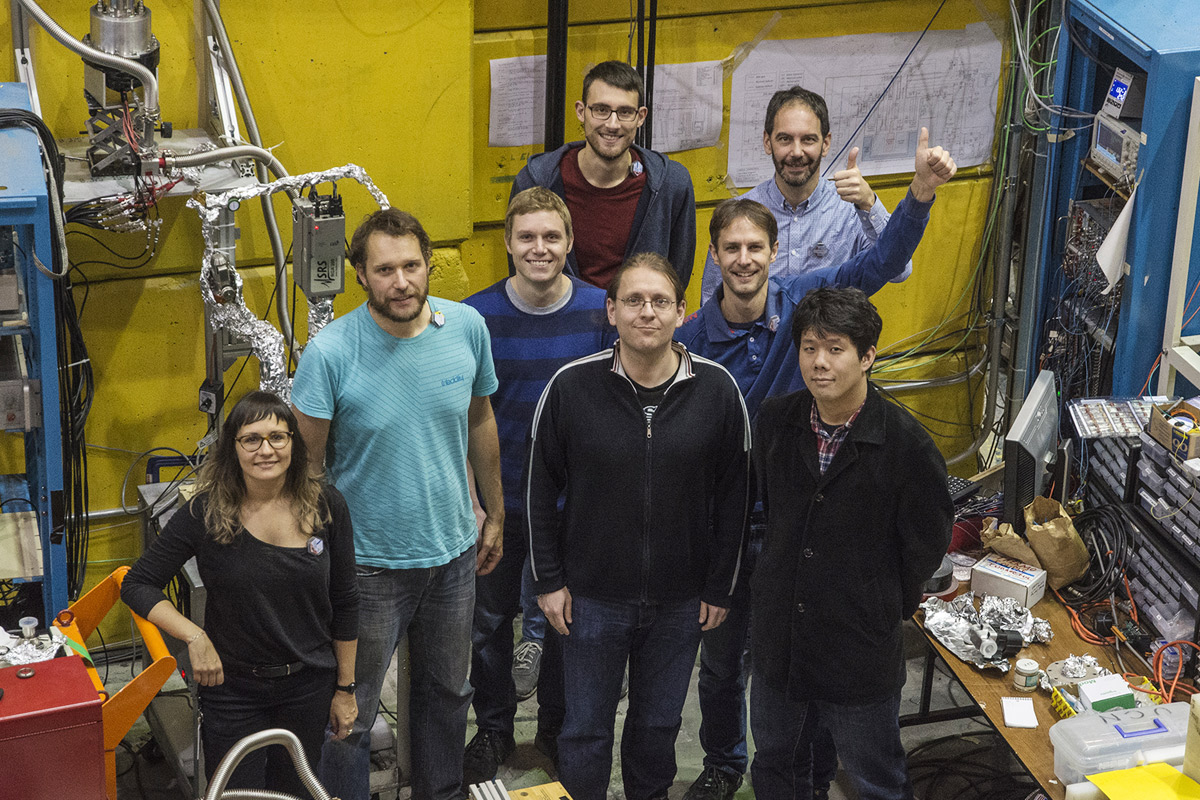
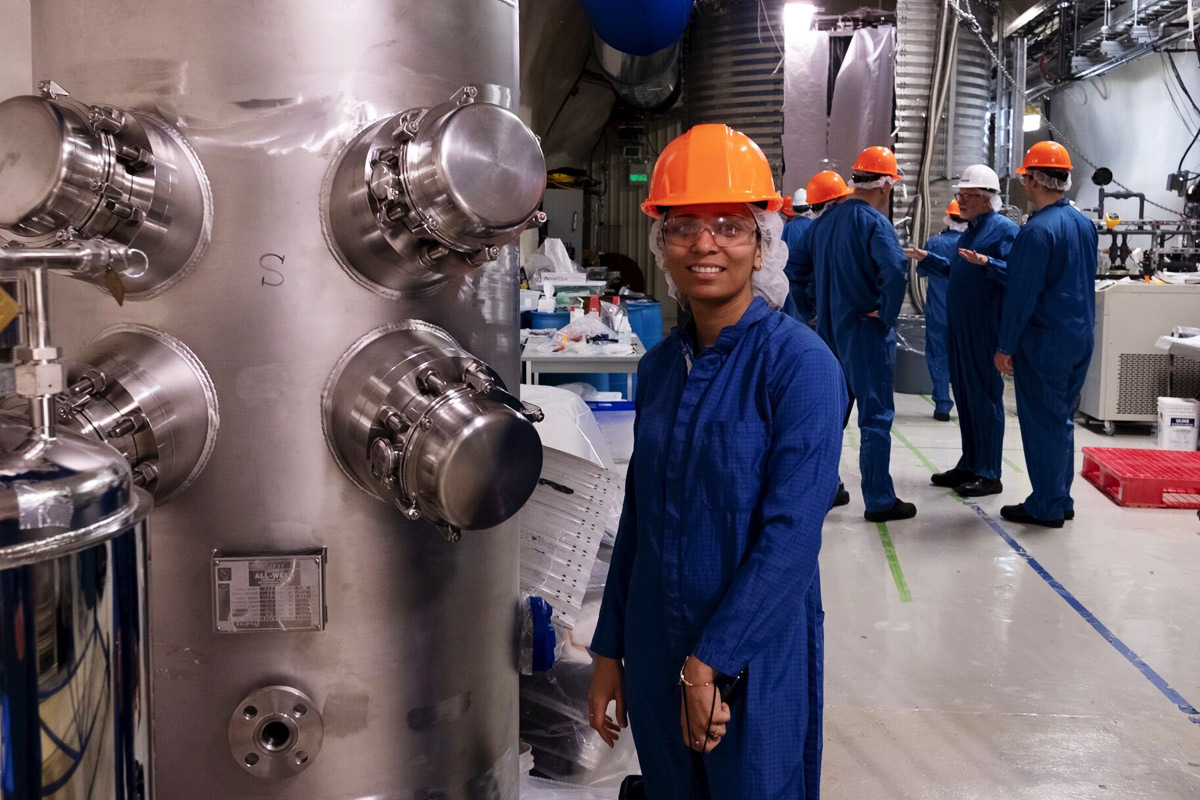
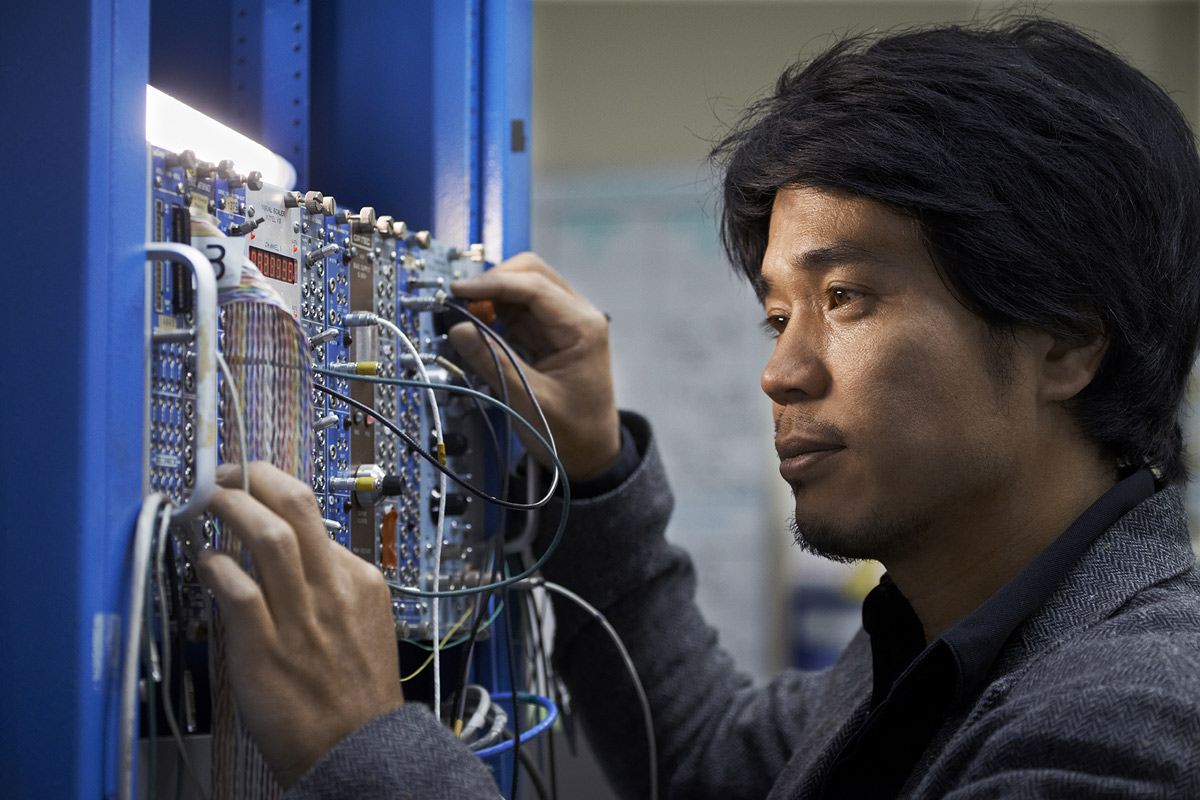
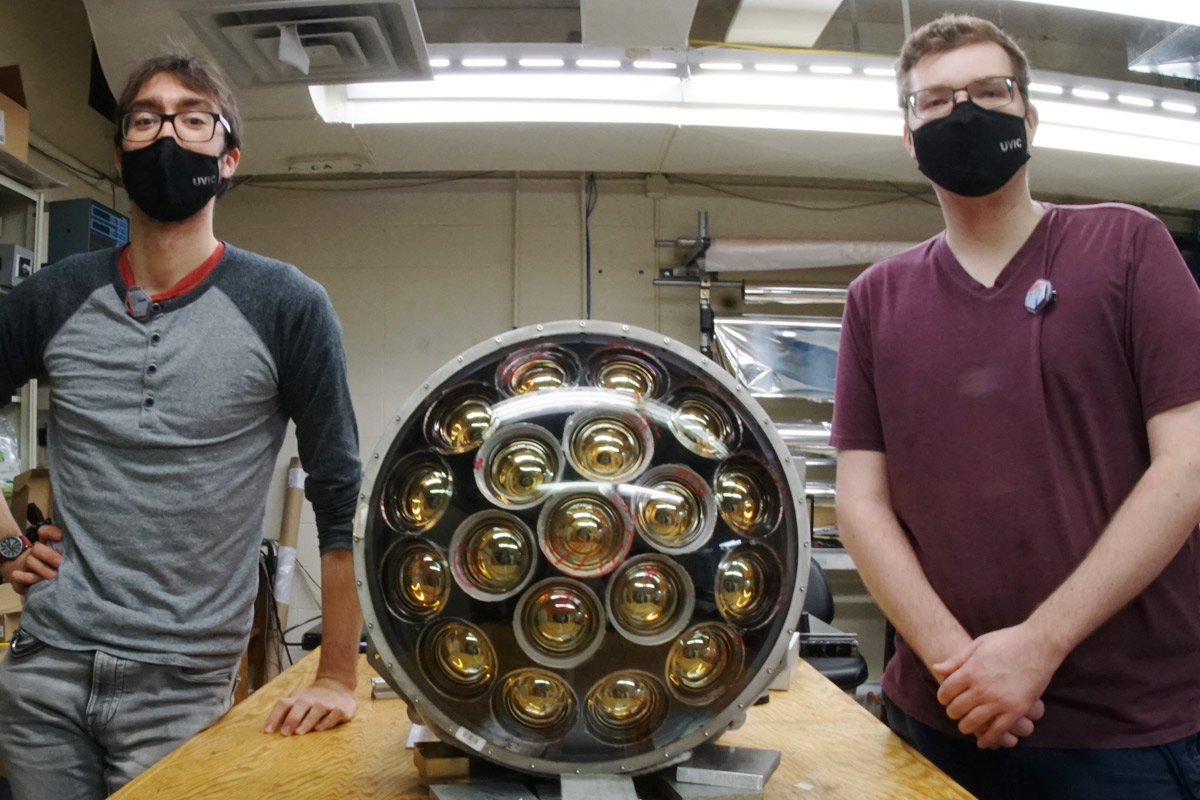